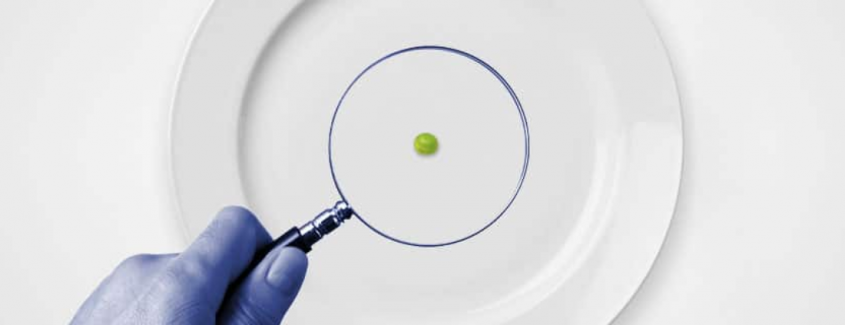
Precis: The high relapse and mortality rates of eating disorders, specifically anorexia nervosa, have provoked great amounts of research into treatment. Recently, genome wide association studies (GWAS) have found exciting evidence of genetic and metabolic predispositions. These new insights may lead to a reconceptualization of anorexia nervosa treatment protocol.
Genetic Research on the Etiology of Eating Disorders
Alessandra Elyse Lane
Grove City College
Eating disorders (ED) have the highest mortality rates of all psychiatric disorders (Watson et al., 2018). Common causes of death for an individual with an ED include cardiovascular complications due to low body weight and suicide (Attia, 2010). These mortality rates have provoked a great amount of research into treatment for these disorders. For several decades, clinicians have believed that eating disorders were triggered by overcontrolling mothers, childhood abuse, low self-esteem, and other environmental and sociocultural factors. While psychosocial factors may be relevant in some cases, new research in genetics suggests that the origins of eating disorders are more physiological and heritable than previously thought.
Anorexia nervosa (AN) is one of the most common types of eating disorders (Watson et al., 2018). Research is finding significant genetic and metabolic effects which may be genetic risk factors for AN development. Learning of a genetic predisposition to AN may lead to a reconceptualization of AN treatment protocol. Although researchers have known of a genetic basis for AN since 1989, knowledge of the genetic role in AN is rapidly expanding recently due to advances in technology (Breithaupt, Hübel, & Bulik, 2018). Genome-wide association studies (GWAS) on AN are emerging, but at this point the literature on other ED types is lacking. The purpose of this paper is to summarize the existing research exploring the role of genetics in the etiology of AN.
A brief history of eating disorders displays their complexity. AN, characterized by abnormal dietary restraint, an intense fear of gaining weight, and severe restriction of food intake has been explored sporadically since the late 17th century (Klein & Walsh, 2004; Silverman, 1997). In 1689, the physician Richard Morton described two patients as suffering from what he called “nervous consumption” in the first medical account of AN, Phthisiologia, se Exercitationes de Phthisi (Silverman, 1997). His pharmacotherapeutic treatments included aromatic bags and “bitter medicines sufficiently impregnated with Spirit of salt Armoniack” (Silverman, 1997, p. 11). One of his patients died shortly after, and the other did not experience any benefits whatsoever. Very few patients with this “nervous consumption” were written about for the next 150 years. In the 1850s, Dr. Louis-Victor Marcé in Paris described a patient with symptoms of what would, 15 years later, be described as “anorexic” (Silverman, 1997). This new condition was understood as a psychological, rather than physical, ailment.
In 1873, anorexia was first described in medical literature by Charles Laségue, who was against the vogue force-feeding treatments of the time (Silverman, 1997; Klein & Walsh, 2004). Instead, Laségue recommended quiet, enduring observation until the patient hit rock-bottom, where the physician could then resume authority and treat the patient with “some effectiveness” (Silverman, 1997). In the early twentieth century, medical opinion vacillated between AN being a psychological disorder to it being a pituitary insufficiency. John Ryle (1936) provided a new theory into the AN patient’s motivations, saying that the patient does not abstain from food because of a physiologically suppressed appetite, but from a morbid mental state forbidding the patient to satiate the patient’s hunger. The movement from psychoanalytic theories and methods to an increased interest in sensible, pragmatic therapeutic methods and research on the neuropsychology of anorexia summarize the literature from the 1970s up until the 1990s.
Starting around 1996, twin studies revealed that eating disorders had a strong genetic component. Early family studies revealed that 5% of first-degree relatives of individuals with AN would develop AN or bulimia nervosa themselves (Berrettini, 1996). Twin studies found that when one monozygotic twin had AN, the other twin was significantly more likely to develop AN when compared with dizygotic twins. For instance, Berrettini (1996) found monozygotic twin concordance rates of 55%, compared with dizygotic twin concordance rates of only 5% in a sample of 200 affected sibling pairs. These findings yielded a heritability estimate of about 50% (Berrettini, 1996). This and other twin studies suggested that genetic vulnerabilities may exist for both eating disorders and their underlying traits. However, twin studies were limited by inability to discern what these vulnerabilities might be (Treasure, 1997). Prior to genome-wide association studies, researchers found a clue to a possible genetic vulnerability. Treasure (1997) noted that pigs that had been bred for leanness later developed anorexic-like symptoms when stressed (Treasure, 1997). It was also suggested that genetic vulnerabilities to certain personality traits common in AN patients such as perfectionism and impulsiveness influence AN development.
Many researchers at the time presumed that AN heritability involved a “gene-environment interaction” (Collier et al., 1997, p. 2). Individuals with a genetic predisposition would be more susceptible to sociocultural factors. The popular opinion at the time of the cause of increased prevalence of AN among female adolescents was extreme social pressure to be thin and therefore more socially desirable (Wakeling, 1996).
Today, twin studies still suggest a significant genetic component contributing to eating disorder development, with recent heritability estimates ranging from 48-74% (Yilmaz, Hardaway, & Bulik, 2015). Twin studies describe population variance but what is needed is a way to discern the location of genetic influence. Genome-wide association studies (GWAS) can determine the statistical significance of an association between a trait and single-nucleotide polymorphisms (SNP) which can influence disease risk. These studies also provide information about ancestry and help predict how people will react to different medications. GWAS can also estimate SNP-based heritability, which is the phenotypic variance (the measured variance of a trait) explained by common genetic variants (alterations in the DNA nucleotide sequence) (Breithaupt et al., 2018). GWAS require huge sample sizes in order to have statistical significance. For instance, the Eating Disorders Working Group of the Psychiatric Genomics Consortium (PGC-ED) has sampled more than 100,000 individuals with eating disorders for this purpose (Bulik, Blake, & Austin, 2019).
In 2017, a GWAS run by the PGC-ED found the first genome-wide significant locus on chromosome 12, which was previously associated with type 1 diabetes and rheumatoid arthritis (Duncan et al.). Significant correlations with metabolic phenotypes in chromosome 12 indicate that genetic metabolic factors may affect weight and appetite dysregulation typical of AN. A normal or high body mass index (BMI), leptin, type 2 diabetes, insulin resistance, and insulin glucose were negatively correlated with AN (Duncan et al., 2017; Watson et al., 2018).
Significant genetic correlations between AN and other psychiatric traits such as schizophrenia, major depressive disorder (MDD), anxiety disorders, and obsessive-compulsive disorder (OCD), as well as personality traits such as neuroticism, and educational attainment, were discovered (Duncan et al., 2017; Watson et al., 2018). These positive correlations may help partially explain the comorbidity between AN and mood disorders such as anxiety and MDD (Breithaupt et al., 2018). SNP-based heritability of AN was significant at 0.20, suggesting that genetic similarities contribute to twin-based heritability (Duncan et al., 2017). Eight risk loci have also been identified (Watson et al., 2018). Significant genetic correlations between AN and both psychiatric and metabolic traits like BMI indicate that the disorder has both metabolic and psychological sources. It is possible that starvation triggers these metabolic effects which, in turn, partially contribute to AN pathology (e.g. perception of being fat) in individuals who are genetically susceptible.
Another study by the same authors again found genomic variants influencing body mass. Increasing variants were associated with binge-eating disorder (BED) while decreasing variants were associated with AN. The researchers propose that AN patients may also carry genomic variants predisposing lower bone mineral density, thereby predisposing them to osteopenia which is common in AN patients (Hübel et al., 2019).
SNP-based genetic correlations (SNP-rg) between AN and traits such as physical activity and HDL cholesterol were significant (Watson et al., 2018). There was also a positive correlation with the gene NCAM1 which increases expression when food is restricted. Even though we know this expression is increased, the consequence of this is unknown.
Low BMI has typically been seen as a symptom and result of AN, but generalized Summary-data-based Mendelian Randomization (GSMR) analyses indicated a significant bidirectional relationship (Watson et al., 2018). BMI-lowering alleles may increase the risk of AN, and AN risk-increasing alleles may increase the risk of a low BMI. Metabolic dysregulation may help explain anthropometric traits commonly seen in AN patients, including why it is so difficult for people with AN to maintain a healthy weight after recovery (Watson et al., 2018).
In 2018, another GWAS sought to extend the findings of Duncan et al. (2017) and Watson et al. (2018) by being the first study to examine low-frequency and rare variants of AN. Despite being a well-powered study in order to detect low-frequency variants with large effect sizes, no findings reached genome-wide statistical significance (Huckins et al., 2018). However, three genes had noteworthy associations with metabolic and anthropometric phenotypes. OPCML, associated with waist-to-hip ratio, FAM96A, associated with several metabolic phenotypes, and C6orf10 may be a part of a metabolic predisposition to AN development. C60rf10 approached statistical significance and was particularly interesting as it has previously been associated with childhood obesity (Huckins et al., 2018). It has been found that appetite and satiety dysregulation is important in the development of childhood obesity, as well as AN. The researchers suggest that there may be a shared biological dysregulation between childhood obesity and AN (Huckins et al., 2018).
One of the greatest risk factors for AN is being female. Females have a much greater risk of developing AN than males who only make up an estimated 5-10% of AN cases. Previous research exploring this gender difference emphasized the sociocultural factors such as social pressures to conform to an unrealistically thin ideal as portrayed in the media. Hübel et al. (2018), using the same GWAS data as Duncan et al. (2017) and Watson et al. (2018), explored the possibility of a genetic explanation for this notable gender difference. More specifically, the relationship between body fat percentage and fat-free mass among men and women with AN was examined. Seventy-seven genome-wide significant loci were associated with body fat percentage and 174 were associated with fat-free mass. SNP-based heritability estimates for body fat percentage ranged from 29 to 33% and for fat-free mass between 43 and 51% (Hübel et al., 2018). Neither significantly differed between sexes, however.
After sex-specific analyses, a statistically significant correlation between female body fat percentage and AN was -0.44. For males the correlation was only -0.26, indicating a greater genetic correlation between female body fat percentage and AN than male body fat percentage (Hübel et al., 2018). Partitioned heritability analysis, testing the contribution of different cell-type groups (i.e. central nervous system cell type and adipose tissue), can classify gene types and their association with disease phenotypes (Breithaupt et al., 2018). After partitioned heritability analysis, the researchers found that body fat percentage in females showed a significant enrichment of the central nervous system cell type, while male body fat percentage showed a significant enrichment of adipose tissue, indicating a sex-specific enrichment for body fat percentage (Hübel et al., 2018). Physical activity and fasting insulin in females were significantly associated with AN. Body fat percentage and fat-free mass measured in adulthood did genetically correlate with AN, however it did not in childhood (Hübel et al., 2018). This may indicate age-dependent genetic variations affecting anthropometric traits and points to a genetic metabolic component. This study was limited due to the small number of male AN cases in the GWAS.
GWAS have advanced the field of psychiatric genetics immensely in the past few years. Nevertheless, it does have its limitations. For one, the reliability of psychiatric diagnoses is less than perfect. Diagnoses are made from the DSM-5 criteria and observations. Diagnoses in a psychiatrist’s office may differ from biomarkers of illness and genetics, which may in turn contaminate the results of GWAS (Breithaupt et al., 2018). Furthermore, significant loci, which are positions on a chromosome where a particular gene is, do not identify the genes themselves. They often implicate several genes, which makes identification complicated and is made even more difficult because of small effect sizes (Breithaupt et al., 2018). Polygenicity, which is the effect of many gene variants contributing to disease liability, is usually too small for GWAS to find. New techniques within GWAS are being developed so researchers can assess polygenicity (Breithaupt et al., 2018).
Another issue with GWAS is that they require massive sample sizes in order to find any significant genome-wide loci. This makes recruiting crucially important for the success of GWAS. The Anorexia Nervosa Genetics Initiative (ANGI) is designed to rapidly recruit individuals with AN to create the largest genetic sample of AN genomics so that the data can be used in future research. For Thornton et al., 2018, recruitment occurred via national registers, treatment centers, social media, newspapers, and other forms of advertising online (Thornton et al., 2018). This included posting on well-known ED blogs and recovery pages. All recruits completed online questionnaires and met the DSM-5 criteria for AN. This was an international recruitment and DNA sampling happened differently depending on the country. For example, ANGI in Australia sent blood kits that the participant could take to a local physician’s office or laboratory and then send back, but recruits from Sweden had to go to their local hospitals (Thornton et al., 2018). Results indicate that individuals with AN were most successfully recruited through social media, but controls were recruited best over email.
As previously alluded to, there is evidence of shared heritability between AN and several other psychiatric disorders. For example, attention-deficit/hyperactivity disorder (ADHD) and EDs often co-occur. While their shared etiology is not fully understood, a 2019 study did find genetic associations between the two disorders. Individuals with ADHD were significantly more likely to have an ED, when compared with individuals without ADHD (Yao et al., 2019). This association was found in both sexes. It was found that relatives of individuals with ADHD were also at an increased risk of EDs. The more closely people were related to an individual with ADHD, the greater the risk was; and the size of the associations decreased as the relatedness decreased (Yao et al., 2019). It is noteworthy that there was a stronger genetic association between ADHD and non-AN EDs (.37) than for AN (.14). While the genetic overlap between ADHD and EDs may reflect a general genetic predisposition to psychiatric disorders, this new evidence may also suggest the presence of specific genetic correlations that do not generalize across psychiatric disorders (Yao et al., 2019).
Schizophrenia is also a highly heritable disease with substantial shared genetic risk with AN. Recent research has found that AN patients are significantly more likely to have schizophrenia than individuals without AN (Zhang, Kuja-Halkola, Bulik, & Bergen, 2019). Furthermore, women with AN were twice as likely as men to also have a diagnosis of schizophrenia.
A genetic overlap between AN and OCD has also been found. Patients in this study were divided by their age of disorder onset (Plana et al., 2019). Very-early onset patients were less than 13 years old and early onset patients were from 13 to 18 years old. Results indicate no significant differences in sex or family history of psychiatric disorders between early onset and very-early onset AN and OCD groups (Plana et al., 2019). After conducting a genetic association study, two SNPs of the HTR2A were associated with very-early onset AN and OCD. HTR2A is a gene that encodes serotonin receptors. Its mutation is associated with OCD susceptibility. Finding these two SNPs associated with AN and OCD supports the existence of a genetic overlap between the two psychiatric disorders (Plana et al., 2019).
But genetic research is advancing quickly and studying the genetics of many psychiatric disorders simultaneously can be done. Pettersson et al. (2019) studied the homogeneity of family-based and SNP-based heritability estimates in eight psychiatric disorders, AN among them. All disorders were moderate to highly heritable, but there was a range between the disorders. There was a positive correlation between family-based and SNP-based estimates, which suggests that greater family-based heritability is associated with the greater effect of SNPs (Pettersson et al., 2019). The heritability estimate of AN was 41%, comparable to heritability estimates mentioned earlier in this paper. The researchers note that non-genetic influences also play a substantial role in AN development (Pettersson et al., 2019).
Using genome-wide association data, 17 phenotypes were examined to quantify genetic risk for psychiatric disorders, AN among them, and neurological disorders such as Alzheimer’s disease, epilepsy, and Parkinson’s disease. There was significant sharing between AN, OCD, and schizophrenia (Brainstorm Consortium et al., 2018). For measured cognitive traits, AN, autism spectrum disorder, bipolar disorder, and OCD were positively correlated. This may indicate a connection between early cognitive performance and genetic risk (Brain Consortium et al., 2018). For personality symptoms, AN significantly positively correlated with neuroticism. Consistent with previous GWAS, BMI negatively correlated with AN, suggesting a partial genetic explanation for low BMI in AN patients post recovery (Brain Consortium et al., 2018).
Because of how many neurological and psychiatric disorders were examined, a unique facet of this study is the fact that significant degree of overlap in genetic risk was quantified. Overall there was a greater amount of genetic overlap between psychiatric disorders than neurological disorders (Brain Consortium et al., 2018). This suggests two things. First, that distinctions clinicians make between psychiatric disorders may not reflect the underlying genetic similarity. If true, current diagnostic criteria almost certainly do not reflect the specific genetic factors of psychiatric disorders as currently described. A reconceptualization of psychiatric disorder diagnostics may be necessary as additional genetic research is published. Second, in contrast to the intertwined genetics of psychiatric disorders, neurological disorders are more genetically distinct from one another (Brain Consortium et al., 2018). Because neurological disorders exhibit less genetic overlap, it is understandable that the diagnostic criteria for neurological disorders includes greater specificity as well.
This paper summarizes the current literature on the genetic etiology of AN. GWAS have supported what twin studies have demonstrated for several decades, that AN is highly heritable. AN exhibits genetic risk factors including the first significant genome-wide locus found on chromosome 12, suggesting that individuals who later develop AN may have a genetic metabo-psychiatric predisposition. Individuals with AN may also be genetically predisposed to have certain anthropometric traits, including a negative correlation with BMI, insulin resistance, and insulin glucose. AN is also positively correlated with fasting insulin and physical activity. Personality factors like neuroticism and educational attainment, as well as psychiatric disorders like schizophrenia, OCD and ADHD positively genetically correlate with AN. Genetic research is rapidly advancing and is encouraging a reconceptualization of AN as a metabo-psychiatric illness influenced by genetic risk factors.
References
Attia, E. (2010). Anorexia nervosa: Current status and future directions. Annual Review of Medicine 61, 425-435. doi: 10.1146/annurev.med.050208.200745
Berrettini, W. (1996). Genetic approaches to anorexia nervosa. Biological Psychiatry 39(7), 503. doi: 10.1016/0006-3223(96)83965-5
Brain Consortium, Antilla, V., Bulik-Sullivan, B., Finucane, H., Walters, R., Bras, J., & Murray, (2018). Analysis of shared heritability in common disorders of the brain. Science 360, 1-12. doi: 10.1126/science.aap8757
Breithaupt, L., Hubel, C., & Bulik, C. (2018). Updates on genome-wide association findings in eating disorders and future application to precision medicine. Current Neuropharmacology 16, 11012-1110. Retrieved from: https://doi.org/10.2174/1570159X16666180222163450
Bulik, C., Blake, L., & Austin, J. (2019). Genetics of eating disorders: What the clinician needs to know. Psychiatric Clinics of North America 42(1), 59-73. doi: 10.1016/j.psc.2018.10.007
Collier, D., Arranz, M., Li, T., Mupita, D., Brown, N., & Treasure, J. (1997). Association between 5-HT2A gene promoter polymorphism and anorexia. The Lancet 350(9075), 412. doi: 10.1016/S0140-6736(05)64135-9
Duncan, L., Yilmaz, Z., Gaspar, H., Walters, R., Goldstein, J., Anttila, V., Bulik-Sullivan, B.,Ripke, S., Eating Disorders Working Group of the Psychiatric Genomics Consortium, Thornton, L., Hinney, A., Daly, M., Sullivan, P., Zeggini, E., Breen, G., & Bulik, C. (2017). Significant locus and metabolic genetic correlations revealed in genome-wide association study of anorexia nervosa. The American Journal of Psychiatry 174(9), 850-858. Retrieved from: http://dx.doi.org/10.1176/appi.ajp.2017.16121402
Hübel, C., Gaspar, H., Coleman, J., Finucane, H., Purves, K., Hanscombe, K., Prokopenko, I.,Graff, M., Ngwa, J., Workalemahu, T., O’Reilly, P., Bulik, C., & Breen, G. (2018). Genomics of body fat percentage may contribute to sex bias in anorexia nervosa. American Journal of Medical Genetics, 1-11. doi: 10.1002/ajmg.b.32709
Hübel, C., Gaspar, H., Coleman, J., Choi, S., Purves, K., Hamscombe, K., Hagenaars, S., O’Reilly, P., Bulik, C., & Breen, G. (2019). 66 Polygenic risk scoring in eating disorders identifies metabolic and anthropometric associations. European Neuropsychopharmacology 29(5), 96-97. doi: 10.1016/j.euroneuro.2019.07.207
Huckins, L., Hatzikotoulas, K., Southam, L., Thornton, L., Steinberg, J., Aguilera-McKay, F.,Treasure, J., Schmidt, U., Gunasinghe, C., Romero, A., Curtis, C., Rhodes, D., Moens, J., Kalsi, G., Dempster, D., Leung, R., Keohane, A., Burghardt, R., Ehrlich, S., Hebebrand, J., …Zeggini, E. (2018). Investigation of common, low-frequency and rare genome-wide variation in anorexia nervosa. Molecular Psychiatry 23, 1169-1180. doi: 10.1038/mp.2017.88
Klein, D., & Walsh, B. (2004). Eating disorders: Clinical features and pathophysiology. Physiology & Behavior 81, 359-374. doi: 10/1016/j.physbeh.2004.02.009
Pettersson, E., Lichtenstein, P., Larsson, H., Song, J., Attention Deficit/Hyperactivity Disorder
Working Group of the iPSYCH-Broad-PGC Consortium, Autism Spectrum Disorder
Working Group of the iPSYCH-Broad-PGC Consortium, Bipolar Disorder Working Group of the PGC, Eating Disorder Working Group of the PGC, Major Depressive Disorder Working Group of the PGC, Obsessive Compulsive Disorders and Tourette Syndrome Working Group of the PGC, Schizophrenia CLOZUK, Substance Use Disorder Working Group of the PGC, Agrawal, A., Borglum, A., Bulik, C., Daly, M., Davis, L., Demontis, D., Edenberg, H., Grove, J., … Polderman, T. (2019). Genetic influences on eight psychiatric disorders based on family data for 4 408 646 full and half-siblings, and genetic data of 333 748 cases and controls. Psychological Medicine, 1-8. doi: 10.1017/S0033291718002039
Plana, M., Torres, T., Rodriguez, N., Boloc, D., Gasso, P., Moreno, E., Lafuente, A., Castro- Fornieles, J., Mas, S., & Lazaro, L. (2019). Genetic variability in the serotoninergic system and age of onset in anorexia nervosa and obsessive-compulsive disorder, Psychiatry Research 271, 554-558. doi: 10.1016/j.psychres.2018.12.019
Ryle, J. (1936). Anorexia Nervosa. The Lancet, 893-899. Retrieved from: www.thelancet.com
Silverman, J. (1997). Anorexia nervosa: Historical perspective on treatment. D. Garner & P. Garfinkel (Eds.), Handbook of Treatment for Eating Disorders (pp. 3-10). New York, NY:The Guilford Press
Thornton, L., Munn-Chernoff, M., Baker, J., Jureus, A., Parker, R., Henders, A., Larsen, J., Petersen, L., Watson, H., Yilmaz, Z., Kirk, K., Gordon, S., Leppa, V., Martin, F., Whiteman, D., Olsen, C., Werge, T., Pedersen, Kaye, W., … Bulik, C. (2018). The anorexia nervosa genetics initiative (ANGI): Overview and methods. Contemporary Clinical Trials 74, 61-69.doi: 10.1016/j.cct.2018.09.015
Treasure, J. (1997). An integration of animal models and genetics into the spectrum of eating disorders. Biological Psychiatry 42(1), 148. doi:10.1016/S0006-3223(97)87499-9
Wakeling, A. (1996). Epidemiology of anorexia nervosa. Psychiatry Research 62, 3-9. doi:S0165-1781(96)02983-6
Watson, H., Yilmaz, L., Thornton, L., Hübel, C., Coleman, J., Gaspar, H., Bryois, J., Leppa, V., Mattheisen, M., Ripke, S., Yao, S., Giusti-Rodrigue, P., Anorexia Nervosa Genetics Initiative, Hanscombe, K., Purves, K., Eating Disorders Working Group of the Psychiatric Genomics Consortium, Alfredsson, L., Ando, T., Andreassen, O., … Bulik, C. (2018). Genome-wide association study identifies eight risk loci and implicates metabo-psychiatric origins for anorexia nervosa. Natural Genetics 51 1207-1214. Retrieved from:https://www.nature.com/articles/s41588-019-0439-2
Yao, S., Kuja-Halkola, R., Martin, J., Lu, Y., Lichtenstein, P., Norring, C., Birgegard, A., Yilmaz, Z, Hübel, C., Watson, H., Baker, J., Almqvist, C., Eating Disorders Working Group of the Psychiatric Genomics Consortium, Thornton, L., Magnusson, P., Bulik, C., & Larsson,
- (2019). Association between attention-deficit/hyperactivity disorder and various eating disorders: A Swedish nationwide population study using multiple genetically informative approaches. Society of Biological Psychiatry 86, 577-586. Retrieved from: www.sobp.org/journal
Yilmaz, Z., Hardaway, A., & Bulik, C. (2015). Genetics and epigenetics of eating disorders. Advances in Genomics and Genetics 5, 131-150. doi: 10.2147/AGG.S55776
Zhang, R., Kuja-Halkola, R., Bulik, C., & Bergen, S. (2019). SU47 risk for schizophrenia in individuals with eating disorders and their relatives. European Neuropsychopharmacology 29(4), 1292-1293. doi: 10.1016/j.euroneuro.2018.08.411